When the Lawrence Livermore National Laboratory announced that it had achieved “fusion ignition” in December 2022, the story made headlines all around the world. For a long time, fusion power has been one of the holy grails of energy research, thanks to its potential to provide almost unlimited quantities of clean energy.
For a very long time, in fact. And as its proponents have a history of over-promising and under-delivering, there is a running joke about fusion energy: “it’s the energy source of the future—always has been, always will be”. Will this time be any different?
What is Fusion Power?
We’re all familiar with nuclear power stations – the first ones were completed in the early 1950s, and they now provide just under 10% of the world’s electricity. But all existing nuclear power plants obtain their energy from nuclear fission: when bombarded with a neutron, a uranium-235 atom splits into two smaller fragments, releasing further neutrons and lots of energy.
Nuclear fusion, on the other hand, is the process that powers the Sun. In fusion, two smaller atoms combine to form a bigger one. Typically, two isotopes of hydrogen are used: deuterium (hydrogen-2) and tritium (hydrogen-3). Under the right conditions, they can fuse together to form helium and one neutron.
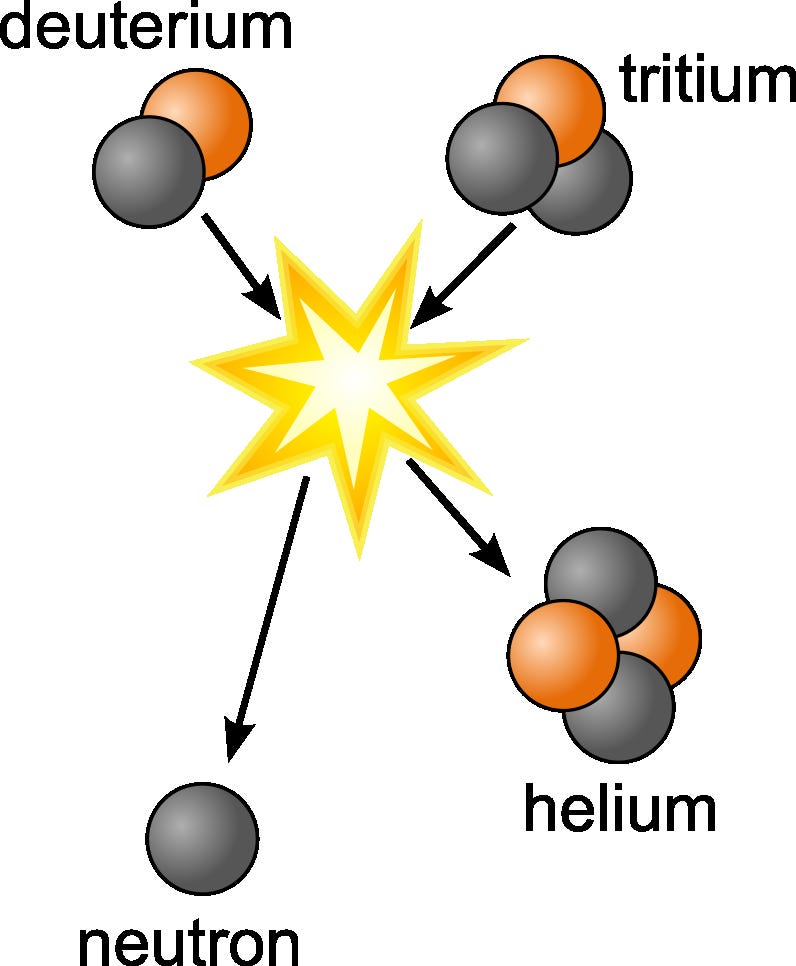
This reaction releases huge quantities of energy (the amount can be calculated using Einstein’s famous equation E = mc2) without any greenhouse gas emissions.
Unfortunately, there’s a catch. In order for the hydrogen isotopes to fuse, they need to be in the fourth state of matter known as plasma. And to convert the hydrogen isotopes into a plasma, you need very high temperatures. In order to generate enough energy in a relatively small volume and at much lower pressure than is found in the Sun, fusion reactors use temperatures of at least 100 million degrees Celsius – ten times hotter than the Sun itself.
Competing Technologies
Research into fusion power has been going on for decades. One of the main research centres has been the Joint European Torus (JET) at Culham in Oxfordshire, which was commissioned in 1983. It is a so-called Tokamak, where a few grams of deuterium and tritium are injected into a massive doughnut-shaped vacuum chamber and then heated.
JET has achieved notable successes: in 1997 it managed to produce 16 MW of fusion power from 24 MW of thermal power, and in 2021 it produced 59 MJ of energy while maintaining a steady 10 MW of fusion power for 5 seconds. However, 59 MJ is only equivalent to the amount of electricity used by a typical household in a single day. Crucially, JET has never reached “fusion ignition” (also referred to as “scientific energy breakeven”) – the key goal of releasing as much energy from the fusion reaction as is required to initiate it.
The National Ignition Facility at the Lawrence Livermore National Laboratory uses a completely approach from JET. Its “inertial confinement” system consists of nearly 200 high-energy lasers surrounding a tiny pellet of deuterium and tritium. This creates conditions that compress the pellet to roughly 1,000 times the density of water while also heating it to more than 100 million degrees Celsius.
This was the technology that finally achieved breakeven last month – 2.05 MJ of energy was used to heat the fuel, and 3.15 MJ of energy was released. In relative terms, that’s an impressive 50% energy gain. However, in absolute terms, the amount of surplus energy was tiny – just 1.1 MJ or around 0.3 kWh. Moreover, that calculation excludes over 500 MJ of energy that the lasers needed in order to deliver the 2.05 MJ input to the target. If you include that, the energy output was only around 0.5% of the total energy input. In other words, we are still a long way from generating net energy in practice.
ITER
ITER, which is the successor to JET, is the world’s biggest fusion experiment, and one of the most ambitious scientific experiments ever undertaken. The project is an international collaboration between the European Union, US, China, Japan, India, Korea and Russia, with the EU financing 45% of the construction phase.
To give an idea of the scale of the project, the scientific platform at Cadarache, France covers 42 hectares, making it one of the largest man-made levelled surfaces in the world. Over 3,000 people are contributing to the civil engineering works. At the heart of the site is the seven-storey building that will house the Tokamak itself. It will be surrounded by another 38 buildings, including an assembly hall for pre-assembly of the Tokamak components, cooling towers and the cryogenics plant that will provide liquid helium to cool the ITER magnets.
The magnets, which are needed to avoid any contact between the hot gas involved in the fusion reaction and the walls of the reaction chamber, will be cooled down to -269 °C to become superconductive. This creates an enormous magnetic cage around the reactor, which is why this approach is called “magnetic confinement fusion”.
Work on site started in 2007, and much of the construction work has been completed, but the project’s timeline has repeatedly slipped. Originally, the plan was to produce the first plasma in 2018, but in 2016 that was pushed back to 2025 and then – due to the pandemic – to a later, as yet unspecified date. Unofficially, internal sources suggest that 2029-31 is more realistic. Deuterium-tritium fusion reactions to produce energy are still scheduled to start in 2035, but when a revised timeline is published, the target date will probably be closer to 2040.
When the time comes, the goal is for ITER to produce around 500 MW of heat for about 7 minutes. This will require 50 MW of input heat, which gives an impressive Q value – the ratio of output heat to input heat – of 10. However, around 300 MW of electricity will be needed to produce the 50 MW of thermal energy, so in practice the energy gain will be much lower. And ITER will not generate any electricity – just heat.
DEMO
If ITER is successful, it will feed into the DEMOnstration power plant, DEMO. The plan is for DEMO to use 80 MW of input heat to produce 2000 MW of thermal energy, with a Q value of 25. This thermal energy will then be used to generate around 750 MW of electricity, which is equivalent to a small conventional power station. DEMO would provide a blueprint for further power plants, but it will not generate electricity at the price and quantities of commercial power plants.
Work on constructing DEMO will start alongside the latter phases of ITER, so the plan was for it to be operational as soon as 2051. In view of the delays to the ITER timeline, however, it seems unlikely that it will be generating any electricity until the mid-2050s at the very earliest.
Can fusion power help us reach net zero?
In view of the above, the ITER/DEMO programme is extremely unlikely to provide the world with significant amounts of fusion power until the 2060s. And with a project as big, complex and ground-breaking as this, success is far from assured. At the very least, further significant delays are to be expected.
Meanwhile, to keep global warming below 1.5°C – as the Paris Agreement calls for – emissions need to be cut by 45% by 2030 and reach net zero by 2050. Magnetic confinement fusion as envisaged by ITER will simply be too late for that.
What about its rival, inertial confinement fusion? After the breakthrough at the Lawrence Livermore National Laboratory, a company founded by a former director of the National Ignition Facility announced that it aims to have a pilot plant based on the technology ready in the early 2030s. That would then lead to “worldwide low-carbon energy” by 2030-50. However, it provided very few details of its plans. Bearing in mind the enormous complexity and costs associated with fusion power, the early end of that date range sounds wildly overoptimistic.
Many experts have also urged people not to raise their expectations too high. In the words of Tony Roulstone, a lecturer in nuclear energy at the University of Cambridge “… this result from NIF is a success of the science – but still a long way from providing useful, abundant, clean energy.”1
Realistically, neither of the main approaches to fusion power is likely to yield the electricity we need to achieve net zero by 2050. They should therefore be left out of the immediate debate on low-carbon energy. Remember that when someone says “we should be focusing on fusion”, the subtext is often “renewables are a waste of time”, and in some cases “we should move on from nuclear fission”. This ignores the fact that only renewables and/or nuclear power can provide the low-carbon electricity that we urgently need now.
Benefits of Fusion Power
If they won’t help us to solve the pressing challenge of climate change, is there any sense in continuing with these exceedingly expensive and resource-intensive fusion power experiments? Should some of the world’s best minds be engaged in what may turn out to be a dead end? These questions are particularly pertinent since it is quite possible that low-carbon sources will already be meeting virtually all our energy needs by the time fusion power becomes available.
Nevertheless, I think there are strong arguments in favour of pursuing the dream of fusion power. Firstly, although the cost is high, it is shared between many countries. Secondly, if we are lucky, it will provide abundant clean energy at some point in the second half of the century. That may be too late for climate change, but it will still be a very valuable supplement to other energy sources.
There’s another reason too. Arguably the main value of the Apollo program was not reaching the Moon as such, but rather its scientific legacy. Similarly, research into fusion power will inevitably lead to important findings that will be of great benefit to other areas of science and technology. The material testing and basic material science that will be done under the umbrella of the International Fusion Materials Irradiation Facility (IFMIF) may prove particularly valuable in that respect.
Achieving fusion ignition has understandably created a renewed buzz about fusion power. It’s an exciting scientific development, and one that deserves to be celebrated. But we must also keep it in perspective: it will be decades until fusion power provides us with meaningful amounts of energy, so it cannot help us in the battle against climate change.
https://www.sciencemediacentre.org/expert-reaction-to-fusion-announcement-from-the-lawrence-livermore-national-laboratory/